Neutrinoless Double Beta Decay
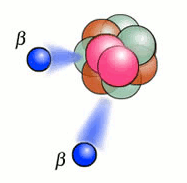
Neutrinos are the second most-abundant particles in the Universe, with an average of about 300 million of them in a cubic metre. They are among the very few truly fundamental particles on which we build our Standard Model of particle physics. Yet, of the particles we know about, neutrinos are the least understood.
In the Standard Model neutrinos are assumed to have exactly zero mass. However, we now have pretty solid evidence that this is actually not true. This evidence comes from the experiments studying a phenomenon known as neutrino oscillations using atmospheric, solar and reactor neutrinos, as well as neutrino beams from particle accelerators.
For a while, physicists have suspected that the Standard Model is part of something bigger: some kind of ultimate theory which would unify all existing interactions, known as Grand Unification Theories (GUT). As it turns out, most of these GUT theories require a small but non-zero neutrino mass.
There are a couple of questions that are absolutely fundamental for our understanding of neutrinos, and that neutrino oscillation cannot answer:
- What is the absolute value of neutrino mass?
- Why are neutrinos so much lighter than the other fermions in the Standard Model?
It is clear that we have to answer the first question. But the second question might be even more fundamental. The answer to this question may be found if we can understand whether the neutrino is a Majorana particle, i.e. identical to its own antiparticle, unlike all the other fermions of the Standard Model that are Dirac particles which have their distinct anti-particle partners.
Experiments which can answer both questions are searching for neutrinoless double beta decay: 0νββ. In fact, 0νββ is the only practical way to understand whether the neutrino is a Majorana or a Dirac particle. It is currently one of the hottest topics in particle physics with many experiments joining the competition. The UCL group has been actively involved in the NEMO-3 and SuperNEMO experiments that are dedicated to search for 0νββ decay.
Double Beta Decay
Double beta decay is a process in which two neutrons are simultaneously transformed into two protons, emitting two electrons and two (anti)neutrinos. (In an ordinary beta decay, only one neutron decays.) This kind of double beta decay (2νββ) is an allowed process in the Standard Model, and has been observed for 12 nuclei. But they are very rare decays, with correspondingly long half-lives. Double beta decay tends to occur in isotopes for which ordinary beta decay is energetically forbidden.
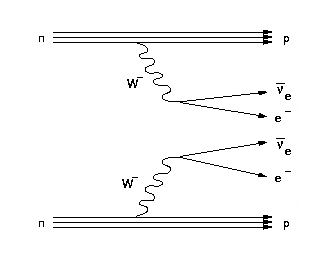
Neutrinoless Double Beta Decay
The most interesting mode of the decay, however, is neutrinoless double beta decay ( 0νββ), in which only two electrons are emitted. This has never been observed, and is only possible if neutrinos are Majorana particles. The process would violate lepton number conservation, and allows the Majorana nature of the neutrino to be directly probed. In fact, observing 0νββ decay is the only practical way to experimentally test the lepton number violation that is a key ingredient of most theories beyond the Standard Model, as well as of many cosmological models that attempt to explain the observed asymmetry between the matter and antimatter in the Universe.
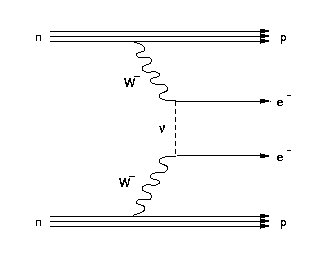
The following is the decay rate equation used for determining the effective Majorana neutrino mass.

where (T1/2)0ν is the measured half life, G0ν is the phase space, which can be calculated, M0ν is the matrix element, which is calculable but has some uncertainties associated with it, and mνe is the effective (Majorana) neutrino mass. As 0νββ decay has not yet been observed, we have only been able to put a lower limit on the half life, leading to a corresponding upper limit on neutrino mass.
Neutrinoless double beta decay has not been observed so far. The current best upper limit on the effective Majorana neutrino mass is < 0.06 - 0.17 eV, from the KamLAND-Zen experiment. The range is due to uncertainties in nuclear matrix element calculations.
This article by Avignone, Elliott and Engel has a more detailed explanation of neutrinoless double beta decay. Boris Kayser has also written a nice explanation of the theory.